Batteries in Human Spaceflight (Part 1)
In this article we are excited to introduce Kush Sutaria, an Aeronautical Engineer with experience working with Li-ion batteries in aircraft and spacecraft. Ever wondered about batteries in space? This is just the article for you, covering all the rules, regulations and realities of how space batteries are engineered to be maximally energy dense and minimally risky.
The Space Environment
Lithium ion (Li-ion) batteries are finding increased use across aerospace, from EVTOLs (electric vertical takeoff and landing aircraft), HAPS (high altitude pseudo satellites) and regional electric aircraft, but one area which people may not know as much about is the use of batteries in spacecraft, particularly human rated spacecraft (ones which carry astronauts).
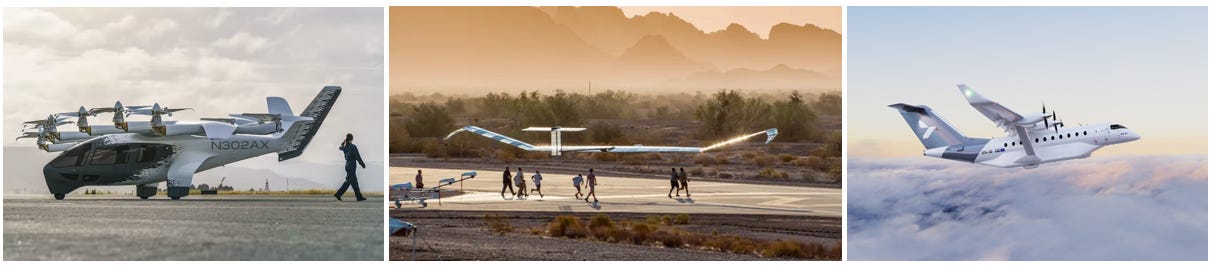
Li-ion batteries are used across all of the main human rated spacecraft including the International Space Station (ISS), SpaceX Dragon and Boeing Starliner. The batteries provide the main electrical power source for when solar panel power is insufficient or if the spacecraft is in darkness. Li-ion batteries also power the spacesuits used for spacewalks on the ISS and which humans will eventually wear when returning to the moon. Astronaut power tools also use Li-ion batteries.
The space environment presents unique challenges when designing batteries, the environment is harsh and testing must be extensive. Some of the key considerations are listed below.
Extreme vibration, acceleration and shock loads during launch and re-entry.
Exposure to vacuum.
Temperature Extremes (-65°C to +125°C in low earth orbit).
High radiation levels (impacts electronic systems such as the BMS)
High specific energy and specific power requirements.
High cycle life required (although the batteries are being cycled once every 90 mins in low earth orbit, voltage windowing is used to extend battery life).
High levels of safety are required, especially as astronauts are onboard.
Thermal runaway propagation is a major concern - the battery must be designed to not allow a single cell thermal runaway to spread to surrounding cells.
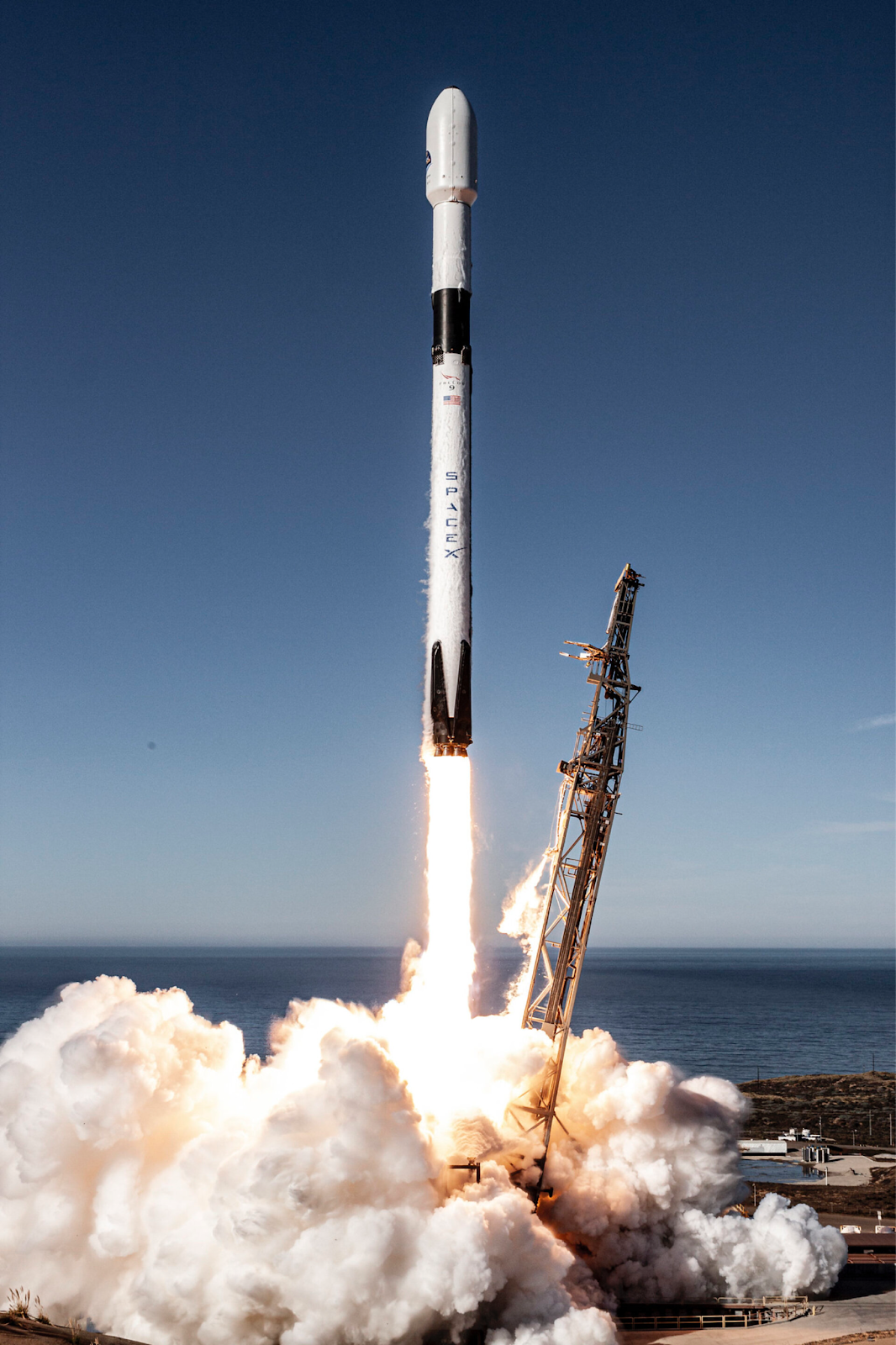
JSC20793 and NASA’s Approach to Battery Safety
The main document covering the battery requirements for crewed spacecraft is JSC20793 and is produced by the Power and Propulsion division at NASA’s Johnson Space Center (JSC). You can find the full document here.
NASA’s approach to battery safety is based on failure tolerance (ie. controlling hazards by using multiple levels of controls). The level of failure tolerance is set based on the voltage/energy of the battery, the higher the voltage/energy, the more levels of control which are needed.
Sometimes, failure tolerance isn’t a viable approach. For example, for controlling against a cell internal short, adding in multiple separators would severely hinder cell performance. In cases like this, DFMR (design for minimum risk) can be used which is based on using mature technology, robust design margins and accepted industry standards across design, manufacture and test.
A key part of reducing the risk of thermal runaway and ensuring battery performance is acceptance testing of cells to ensure uniformity and absence of manufacturing defects. Cells must undergo 100% screening that includes, at minimum, visual inspection of bare cells with shrink wrap removed if present, mass, OCV retention, alternating current (AC) and direct current (DC) resistance. The below flowchart is used to reject outlier cells which are outside of the 6σ range (middle 99.7% of data points) until the lot of cells meets the uniformity criteria.
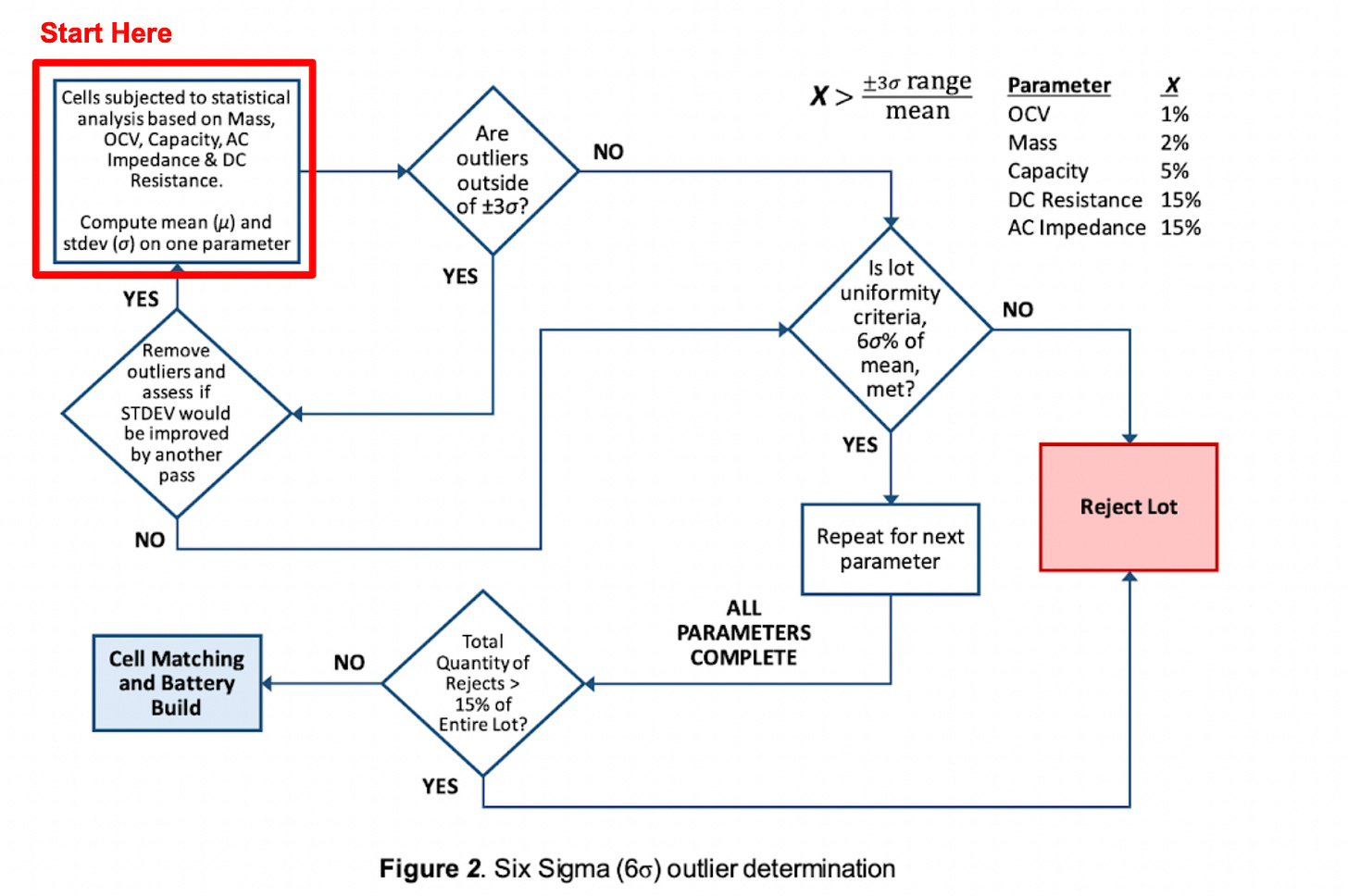
Cell Strategic Reserve
The process for purchasing, shipping and acceptance testing large lots of cells can be cumbersome and a financial and logistical burden on space programs. Supply chain issues can also mean risks to programs in getting their cells on time. In order to help with this, NASA operates a strategic reserve of acceptance tested cells available for purchase to space programs. These cells have been proven as safe designs and have been acceptance tested to ensure the uniformity of the lots and absence of defects. They also come with a full papertrail. Currently only 18650 cells are stocked, but there are plans to expand to 21700s in the future. Prices are around $36/cell for the 18650s. For reference, 18650s usually go for about $4/cell if bought from the manufacturer or off the shelf. The strategic reserve also mitigates the risk of space programs making mistakes in the cell screening process, which can cause schedule and budget slips.
Passive Propagation Resistance
Another key part of NASA’s approach to battery safety is ensuring passive propagation resistance to thermal runaway. We can try and reduce the likelihood of thermal runaway by screening cells, implementing BMS protection features and operating the cells within their limits, but latent defects can always exist in cells from the manufacturing process which could cause an internal short. This means our battery pack must be designed to reduce the severity of a single cell thermal runaway. The runaway should not spread to surrounding cells causing a chain reaction.
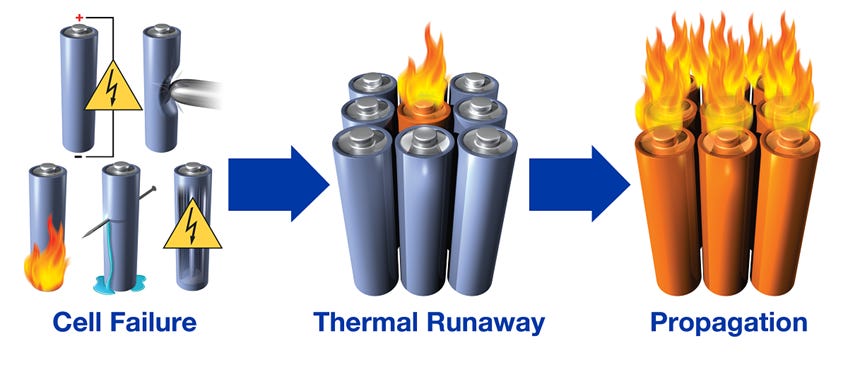
The key design ideas to reducing the severity of a cell thermal runaway are to:
Reduce risk of cell can side wall ruptures
A sidewall rupture is where the thermal runaway blows a hole in the side of the cell and products are vented out the side of the cell. As cells are usually stacked next to each other, this means neighboring cells are in direct line of the heat and gases ejected. This heat could cause the neighboring cell to now go into thermal runaway, causing a chain reaction. We need to pick cells which are less prone to sidewall ruptures and provide material between cells to act as a barrier to any sidewall rupture. Cell designs are extensively tested to assess their propensity to sidewall rupture and destructive physical analysis (DPA) is used to look for defects in cell designs which could cause sidewall rupture.
Sidewall rupture. Image source
Provide adequate cell spacing & heat dissipation
Direct contact between cells without heat dissipation paths will make propagation highly likely, especially if there is a sidewall rupture. We need to space cells out and provide paths for the heat to dissipate away.
NASA design which has an Aluminum honeycomb between the cells. This provides material between the cells and acts as a heat sink. Image source
Individually fuse parallel cells
NASA recommends placing a fuse on the negative side of the cell rated to 200% of the cell design load. The risk is that a thermal runaway cell could become an external short to adjacent parallel cells. As cell vents are usually on the positive cap of the cell, any debris ejected from the top of the cell could bypass the fuse if the fuse is on the top of the cell, hence the recommendation to fuse on the negative side.
Tesla battery packs with wire bonds on each cell acting as fuses. Image source
Protect the adjacent cells from the hot thermal runaway cell ejecta (solids, liquids, and gases).
Thermal runaway ejecta is electrically conductive so could cause a short. We want to avoid direct pathways from one cell to the next.
Prevent flames and sparks from exiting the battery enclosure.
An Aluminium battery pack design which combines structural and heat dissipative features into one part while maintaining cell spacing. The heat sink spreads heat away from cells and each cell has a mica and shrink sleeve insulating layer to avoid the cell heating up too much. You can find more info here in this presentation.
To be continued…
Now that we have a good idea of NASA’s approach to battery design and the key design challenges, in the next article we will explore the specific batteries used on the International Space Station, Spacesuits and Commercial space vehicles. Watch this space!